
Device reveals how drugs affect brain activity in real time
Research with the device may help develop new treatments for neurological diseases and mental health disorders.Media Contact: Leila Gray - 475-9809, leilag@uw.edu
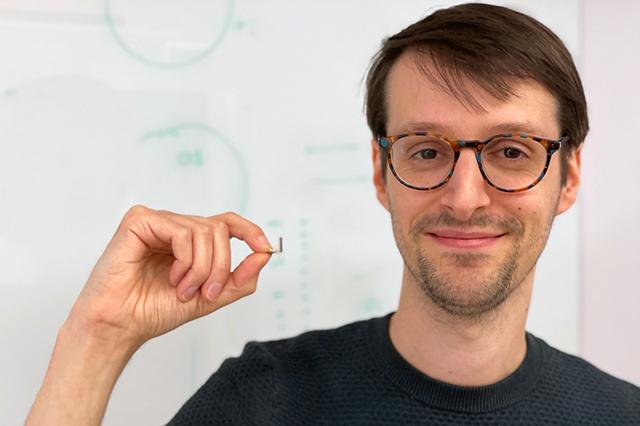
Scientists have developed a tiny device that can deliver drugs directly to a specific spot in a mouse’s brain and show how those drugs affect brain cell activity — all while the mouse is awake and moving freely.
The device is so small and light, about the weight of a paperclip, that the mouse can behave normally while wearing it. That unobtrusiveness allows researchers to study how changes in brain activity influence movement and behavior.
“With this device, we can see what a drug is doing at a specific place in the brain at specific moment in time and see its effect on behavior,” said Sean C. Piantadosi, a postdoctoral fellow in the Department of Pharmacology at the University of Washington School of Medicine in Seattle.
An article describing the new research tool was published in the May 7 print issue of the CELL Press journal, Neuron.
Piantadosi is the co-first author with Min-Kyu Lee and Mingzheng Wu, postdoctoral fellows at Northwestern University. The study was funded by the National Institute of Health’s Brain Initiative.
The study’s goal was to help scientists better understand how drugs and other chemicals interact with brain cells and circuits. This information could lead to better treatments for neurological diseases and mental health disorders, said John Rogers, professor of materials science and engineering, biomedical engineering and neurological surgery at Northwestern.
Rogers and Michael Bruchas, professor of anesthesiology, pharmacology and bioengineering at the UW School of Medicine, were the senior co-authors of the research paper. Bruchas is an investigator at the UW Center for the Neurobiology of Addiction, Pain and Emotion.
The device has two main parts: a tiny pump and a light sensor, both connected to a thin probe that is easily threaded into any area of the brain. It consists of a glass fiber that transmits light and a flexible tube to deliver drugs. The lightweight, battery-free system is powered by radio waves.
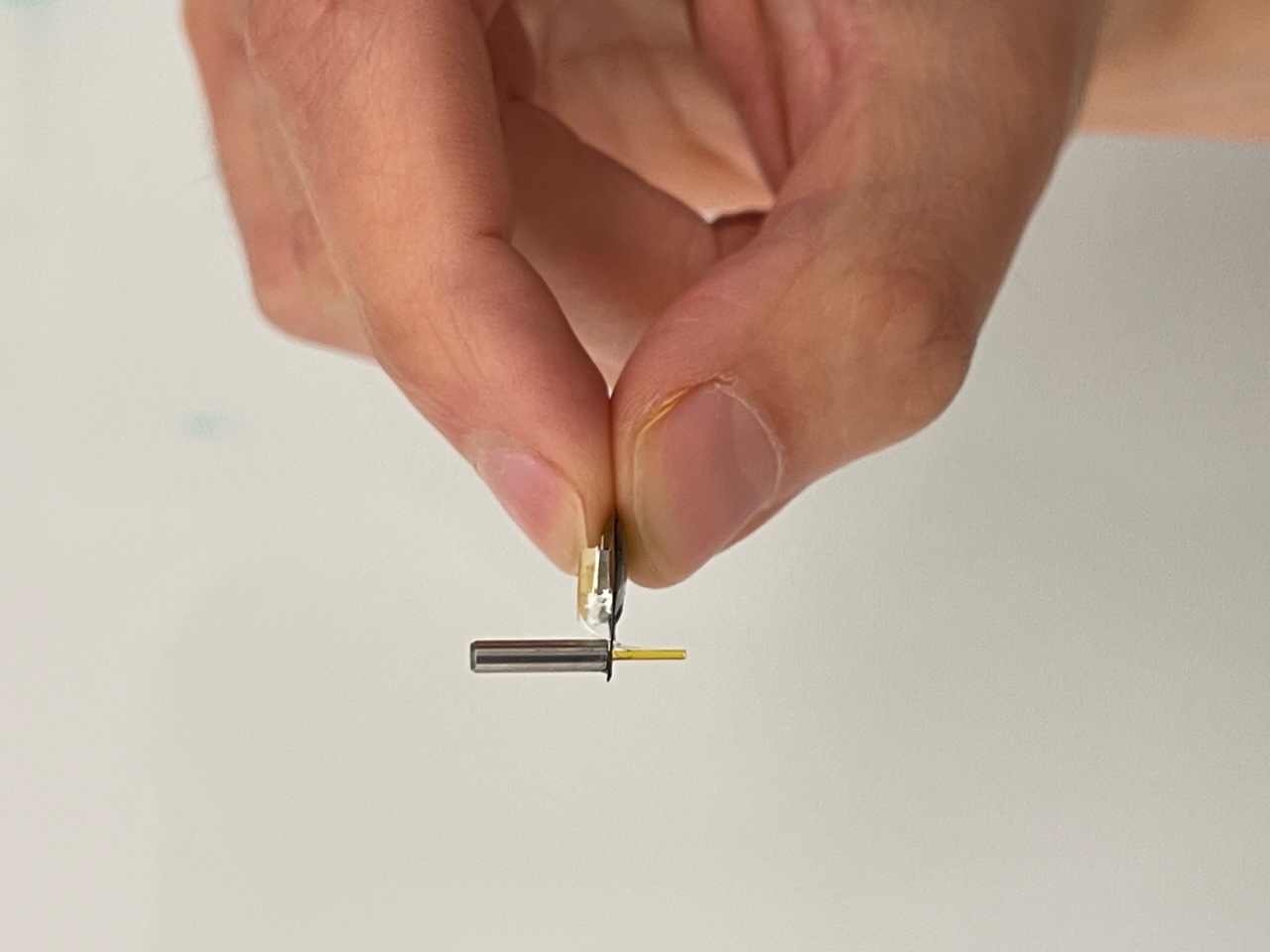
“The advantage of this system is that the mouse can go about its usual activities while wearing the device,” Rogers said. “That makes it much better for observing how neuronal activity affects behavior.”
A key challenge was to ensure the device introduced drugs in a consistent, reliable way, said Min-Kyu Lee, who is training as a researcher in the field of neuro rehabilitation and spinal cord injury.
“By carefully designing the openings of the outlets of the fluid channel,” he said, “we were able to deliver the drugs exactly in the concentration where we wanted.”
Before using the device, the UW researchers genetically engineered the mouse’s brain cells to produce proteins that fluoresce when activated. One such protein, for example, glows when a brain cell fires; another lights up when a chemical signal activates it, for instance, via a neurotransmitter from a different part of the brain.
“This technology brings a new level of precision to studying brain function,” said Mingzheng Wu. “We can precisely control both the location and timing of drug delivery while simultaneously recording neuronal responses.”
To run an experiment, the researchers insert the probe into the part of the brain they want to study. They then use a radio signal to turn on the pump to make it instill a minute amount of a drug or other chemical compound into the target region. Then the light sensor sends LED light down the optical fiber to prompt fluorescent proteins on activated neurons to glow. The light from the fluorescing protein travels back up the optical fiber where it is detected and amplified for measurement.
In one test described in the paper, the team inserted the probe into a cortical brain region that controls movement on one side of a mouse’s body. After the researchers infused a stimulant drug, the targeted brain cells lit up and the mouse began turning in circles — evidence that the drug affected those specific neurons. When a second drug was delivered to stop the neurons from firing, the glowing stopped, and the mouse stopped turning in circles.
“One of the biggest challenges in neuropharmacology has been being able to tell whether a drug you administered systemically is having its effect by acting on the area of the brain you’re studying or through effects it has on other parts of the brain,” said Bruchas. “Now, we can isolate a drug’s effect in a specific region of the brain and on behavior in real time, which we’ve never been able to do before.”
Support for this research came from the National Institute on Neurological Disease and Stroke’s Brain Initiative (1U01NS131406), National Institutes of Mental Health (R01MH112355, R01MH111520), the National Institute of General Medical Sciences (T32GM085270-12), the National Institute on Drug Abuse (R33DA051489, P30DA048736-05, K99DA0623238, F31DA059186-02), and the National Institutes of Health (R42MH116526)
Written by Michael McCarthy.
For details about UW Medicine, please visit http://uwmedicine.org/about.